With the development of the economy, my country’s scrap steel resources are gradually increasing. It is expected that by 2030, my country’s scrap steel resources will increase from the current 200 million tons to 300 million tons. Under the guidance of national energy conservation and consumption reduction, the reuse of scrap steel resources is particularly important. Electric furnace steelmaking uses scrap steel as the main raw material and has its unique development advantages. At present, magnesia carbon bricks are commonly used as melt pool bricks and slag line bricks in electric furnaces. The performance of magnesia carbon bricks is a key factor in the service life of electric furnaces, and the type of antioxidant added is crucial to the performance of magnesia carbon bricks. This paper studies the effects of the addition of antioxidant boron carbide, aluminum powder, silicon powder and the composite addition of aluminum powder and silicon powder on the volume density, apparent porosity, normal temperature compressive strength, high temperature flexural strength, heating permanent line change, and rotational slag resistance of magnesia carbon bricks impact.
1 Experiment
The main raw materials used in the experiment are 97.2 fused magnesia, -196 flake graphite, liquid resin, and high-temperature asphalt.
Ingredients according to the experimental recipe. Pour the large particles into the mixer and mix dry for 2 minutes. Slowly add liquid resin and mix wet for 2 to 3 minutes. Then add graphite and mix for 5 minutes. Finally, add fine powder and mix for 20 to 25 minutes. After discharging, press with 1000 t friction. The bricks are pressed and formed by machine and dried in an oven at 200°C for 8 hours. The apparent porosity, bulk density, compressive strength, high temperature flexural strength, rotary method slag resistance, and microstructure analysis of the samples were tested in accordance with national standards. Among them, the slag used in the rotary slag resistance test is the final slag of a certain company’s electric furnace, and its chemical composition is shown in Table.

2 Results and discussion
2.1 Bulk density, normal temperature compressive strength and apparent porosity
The bulk density, normal temperature compressive strength and apparent porosity of the sample are shown in Figure 1. It can be seen from Figure 1 that the addition of boron carbide, aluminum powder and silicon powder causes the volume density and normal temperature pressure strength of the sample to decrease, and the apparent porosity to increase. The sample with silicon powder added has the lowest volume density and the highest apparent porosity; the sample with aluminum powder has the lowest temperature and compressive strength. This is because: the volume density of the antioxidant elemental silicon < the volume density of boron carbide < the volume density of elemental aluminum < the volume density of fused magnesite; the porosity of the sample increases, the solid-solid bonding inside the test weakens, and the normal temperature resistance The compressive strength is reduced.
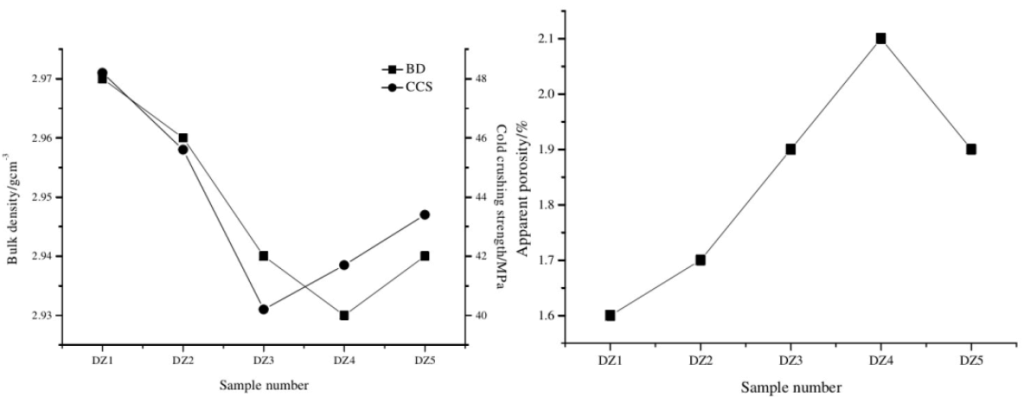
2.2 High temperature flexural strength
The high temperature flexural strength of the sample is shown in Figure 2. It can be seen from Figure 2 that the addition of boron carbide, aluminum powder and silicon powder is beneficial to improving the high-temperature flexural strength of the sample, and the high-temperature flexural strength of the sample is the highest when aluminum powder and silicon powder are added in combination. This is because:
1) In the sample with the antioxidant boron carbide added, the boron carbide preferentially oxidizes C, which greatly reduces the oxygen partial pressure PO2 inside the material, which protects C from being oxidized in large quantities; at the same time, after boron carbide is oxidized, it generates Liquid phase B2O3 forms a liquid film on the surface of the sample, blocking the pores of the material, reducing the diffusion rate of O2 on the surface of the sample, thereby reducing the degree of carbon oxidation; in addition, liquid B2O3 has good wettability to MgO, The two easily react to form trimagnesium borate (3MgO·B2O3), and trimagnesium borate can form a dense protective layer, which can further seal the pores on the surface of magnesia carbon bricks, prevent the intrusion of O2, and thus protect the oxidation of carbon [5]. Boron carbide prevents the oxidation of carbon in magnesia carbon bricks through the above methods, reduces the pores left by carbon oxidation at high temperatures, and strengthens the solid-solid bonding at high temperatures, thereby improving the high temperature flexural strength of magnesia carbon bricks.
2) When magnesia-carbon bricks are heated, the antioxidant aluminum powder reacts with C and CO to form carbides, and re-aggregates C to eventually generate high-melting point substances such as Al4C3, Al2O3, MA, etc., and subsequently produce volume expansion, making the bricks The body is densified to form a ceramic bond, thereby improving the high-temperature strength of the sample.
3) When silicon powder is at 600°C or 1000°C, C reacts with oxygen preferentially to generate SiO(g) and SiO2(s). The generated SiO2 blocks some pores of the material, effectively improving the anti-oxidation performance of magnesia-carbon bricks. As the temperature increases, SiO2(s) reacts with MgO to form M2S with a high melting point, which is accompanied by a certain volume expansion, resulting in densification of the material, thus improving the high-temperature strength of magnesia-carbon bricks.
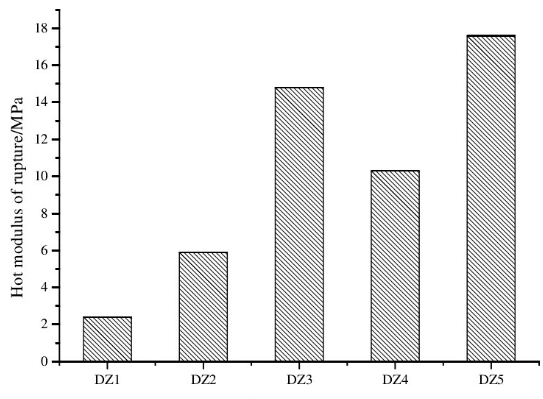
2.3 Line changes after burning
The linear change rate of the sample after burning at 1600℃ is shown in Figure 3. It can be seen from Figure 3 that all samples expanded; compared with the samples without antioxidants, the expansion of the samples with boron carbide and silicon powder was reduced, and the expansion of the samples with aluminum powder and aluminum-silicon composite powder was reduced. The expansion increased, and the expansion of the sample with added aluminum powder was the largest. When boron carbide is added to the sample, liquid phase B2O3 and trimagnesium borate (3MgO·B2O3) are generated after boron carbide is oxidized, both of which promote the sintering of the sample, so the post-sintered expansion of the sample is reduced. For samples with added silicon powder, the silicon powder is oxidized to SiO2 and then forms a glass phase with impurity components, which promotes the sintering of the sample, so the post-sintered expansion of the sample is reduced. For the sample with aluminum powder added, the aluminum powder reacts with MgO after oxidation to form MA and generates volume expansion, so the post-burning expansion of the sample increases.
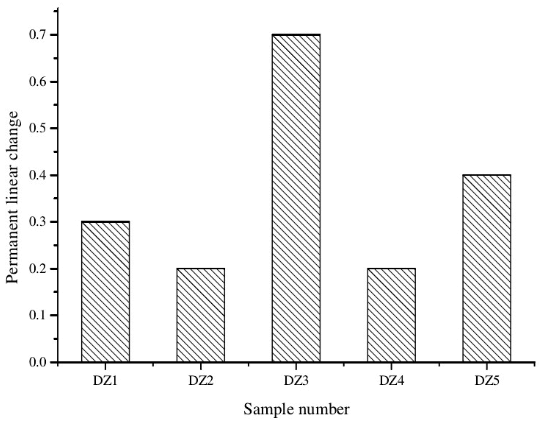
2.4 Slag resistance performance
The photo of the sample after 60 minutes of rotational slag resistance test is shown in Figure 4. It can be seen from Figure 4 that compared with sample DZ1 without antioxidant, the erosion resistance of the sample with boron carbide is significantly reduced; the erosion resistance of the sample with aluminum powder, silicon powder and aluminum-silicon composite powder is not obvious. Variety. The low-melting phase B2O3 and trimagnesium borate (3MgO·B2O3) generated after the oxidation of boron carbide reduce the erosion resistance of the sample; and after adding aluminum powder, silicon powder and aluminum-silicon composite powder, high temperature is generated inside the sample at high temperature. For the melting point phases MA, M2S, and MA and M2S phases, the solid-solid bonding inside the sample did not decrease, and the high-temperature erosion resistance of the sample did not change significantly.
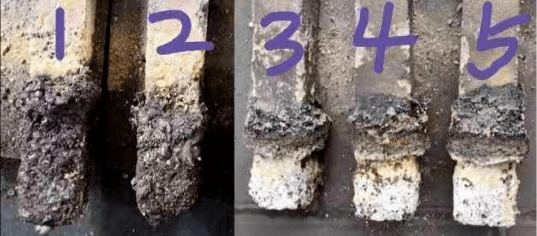
Fe element tracking was performed on the DZ1, DZ2, DZ3, DZ4, and DZ5 samples and it was found that the depths of the samples corroded by molten slag were 1.8mm, 2.7mm, 3.6mm, 2.5mm, and 3.4mm respectively. It can be seen that with the addition of antioxidant boron carbide, aluminum powder, silicon powder and aluminum-silicon composite powder, the depth of corrosion of the sample by the slag increases, and when the amount of aluminum powder added is 3%, the slag of the sample The maximum erosion depth is 3.6mm. This is because the electric furnace slag contains relatively high amounts of CaO and SiO2, and the CaO and SiO2 in the slag react preferentially with the Al2O3 and MgO in the electric furnace magnesia carbon bricks to form anorthosite (2CaO·Al2O3·SiO2) and forsterite. (2CaO·MgO·SiO2) and other low melting point substances, thereby strengthening the erosion of magnesia carbon bricks.
3 Conclusion
(1) When aluminum powder and silicon powder are added in compound, the high-temperature flexural strength of the sample reaches a maximum of 17.6MPa.
(2) The introduction of antioxidant boron carbide and silicon powder into the sample causes micro-expansion, which is beneficial to improving the high-temperature volume stability of the sample.
(3) The samples added with silicon powder have good comprehensive properties.